A Fast, Inexpensive DNA Sequencer In Every Doctor's Office?
By Chuck Seegert, Ph.D.
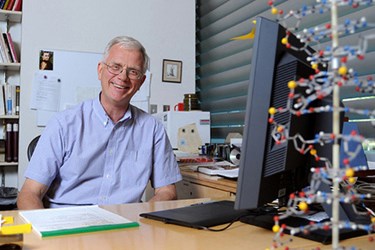
Using conventional computer chip fabrication methods, researchers from Arizona State University’s Biodesign Institute and IBM’s T.J. Watson Research Center have developed a proof-of-concept device that rapidly reads DNA. The technology could one day enable a patient’s genome to be read during a routine doctor’s visit, guiding treatment decisions.
Each individual’s genome is unique, and differences in its makeup determine how they will respond to drugs and other treatments. What may work very well for one person may cause a deadly allergic reaction in someone else. The ability to rapidly examine a person’s genome may allow these differences to be identified up front, eliminating treatment mistakes and enabling more effective, personalized medical treatment sooner.
This is the vision of the combined ASU-IBM research team, according to a recent article from ASU News.
“Our goal is to put cheap, simple and powerful DNA and protein diagnostic devices into every single doctor’s office,” said Stuart Lindsay, an ASU physics professor and director of the Biodesign Institute’s Center for Single Molecule Biophysics, in the article.
To accomplish this lofty goal, the researchers have looked to rapid, low-cost manufacturing methods currently used in the semiconductor industry. Their prototype device is fashioned on the nanoscale and distinguishes individual chemical bases of DNA (cytosine, guanine, adenosine, and thymine) as they pass through the sensor, like a barcode reader in a grocery store. The device can sense DNA in the nanomolar concentration range, which is even better than the most current DNA sequencing technologies.
To create the precision electrodes with a separation of only two nanometers, the team used thin-film technology to metal coat a two-nanometer separating membrane. To precisely allow the fluids to pass between the layers, they merely made small hole.
“Our approach of defining the gap using a thin layer of dielectric (insulating) material between the electrodes and exposing this gap by drilling a hole through the layers is much easier,” Lindsay said in the article. “What is more, the recognition tunneling technology we have developed allows us to make a relatively large gap (of two nanometers) compared to the much smaller gaps required previously for tunnel current read-out (which were less than a single nanometer wide). The ability to use larger gaps for tunneling makes the manufacture of the device much easier and gives DNA molecules room to pass the electrodes.”
The ASU-IBM group is not the only one using this type of approach to DNA analysis. For example, a team at École Polytechnique Fédérale de Lausanne (EPFL) is exploring the possibility of reading DNA through a nanopore with graphene as the conductive sensor material.
Photo credit: Biodesign Institute, Arizona State University