MIT-Created Computer Model Enables Design Of Complex 3D DNA Structures
By Chuck Seegert, Ph.D.
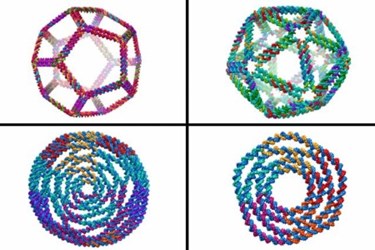
Massachusetts Institute of Technology (MIT) researchers have developed a powerful tool that is capable of designing the most complex DNA geometries ever produced. By accurately predicting the 3D structure of DNA, the design program could allow researchers to develop complex nanoscale biological scaffolds with a minimal amount of experimental effort.
Molecules of DNA are very stable and are easily designed by manipulating base pairs that comprise the strand. The way these base pairs associate and bond with one another then causes the strands to assemble into a unique 3D geometry. Controlling the base pair sequence essentially controls the 3D geometry of the final structure. In the past, this approach has been used to create small 2D structures using a method known as DNA origami.
Taking DNA origami into the 3D space, however, has been a challenge, because predicting final geometries is difficult. A new algorithm developed by MIT researchers may change this, according to a recent article from MIT News. The new program virtually cuts DNA sequences into subcomponents called “multi-way junctions,” and uses staple strands to form complex 3D geometries like cages, tetrahedrons, octahedrons, and more.
The new program is designed to produce 3D DNA structures that, when fabricated, will be compatible with quantitative experiments, according to a recent study published by the team in Nature Communications. The technology could be used to generate 3D structures based on smaller building block motifs, which could then be applied to biomolecular and materials science assemblies.
The vision of the team is to use the DNA molecules as scaffolds and add functional molecules to the DNA backbone, thus creating nanoscale 3D objects, according to the press release. An example of this is replicating the geometry of the Shiga toxin, a protein structure whose shape is especially effective at penetrating cell walls without causing a significant reaction in the cell. If a protein’s shape could be mimicked, the team thinks it may be possible to deliver drugs or various RNA molecules to affect a cell’s function.
“This targeting subunit is very effective at getting into cells, and in a way that does not set off a lot of alarms, or result in its degradation by cellular machinery,” said Mark Bathe, an associate professor of biological engineering, in the MIT article. “With DNA we can build a scaffold for that entry vehicle part and then attach it to other things — cargo like microRNAs, mRNAs, cancer drugs, and other therapeutics.”
While DNA origami shows promise as a new synthetic fabrication method, it also has potential in the diagnostic space. Recently, in an article published on Med Device Online, DNA origami was used to better understand the role of cell signaling in the treatment of cancer.
Image Credit: Top row: 3-D structural predictions generated using CanDo by Stavros Gaitanaros, a researcher in MIT's Laboratory for Computational Biology and Biophysics (LCBB), based on sequence designs provided by Fei Zhang of the Hao Yan Lab at Arizona State University. Bottom row: designs by Keyao Pan (LCBB)/Nature Communcations
Video Credit: Melanie Gonick/MIT