3D-Printed Injection Molding: The Future Of Rapid Prototyping?
By Jim Pomager, Executive Editor
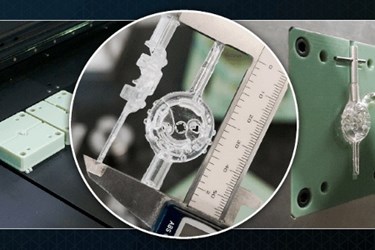
Marrying the speed and economy of 3D printing with the material and accuracy advantages of injection molding
3D printing has fundamentally changed the way medical devices are prototyped and tested. The technique turns out sample parts much more quickly and cheaply than conventional manufacturing methods, enabling designers to significantly shorten iteration cycles and speed time to market for their products. As the cost of equipment has fallen, the technology has improved, and the range of applicable materials has expanded, 3D printers have become a fixture in design departments — even the smallest of medical device companies is investing in these rapid prototyping tools.
But today’s 3D printing technology can carry a product only so far into the development process, primarily due to material constraints. Following a design freeze, it is preferable to build functional prototypes using parent materials — the specified materials intended to be used in the final product — to enable accurate verification and validation testing (as required by the FDA). In many cases, parent materials are incompatible with the current generation of 3D printing technology. Plus, 3D printing can’t always deliver the fidelity or surface finish necessary to satisfy regulatory or internal testing requirements.
As a result, device makers usually turn (back) to traditional manufacturing methods like injection molding (IM) to create their functional prototypes. IM is capable of producing highly accurate plastic parts in parent materials, though the process is not without its own drawbacks. The steel or aluminum molds into which the materials are injected are comparatively expensive, costing thousands or tens of thousands of dollars. (Steel molds can get into the hundreds of thousands.) Manufacturing of these molds takes anywhere from two to six weeks, and that’s before production of prototype parts can even begin. As you might imagine, design changes or mold corrections can be extremely costly, both in time and money.
Over the past year, a hybrid prototyping process has begun to emerge, offering medical device developers the best of both worlds — the speed and economy of 3D printing combined with the material and accuracy advantages of IM. The approach uses 3D-printed molds to generate fully functional, injection-molded prototypes. I recently had the opportunity to discuss the technique with Dan Darst, executive VP and partner at Minneapolis-based product design and development firm Worrell. Darst and his company have been one of the early adopters of the 3D IM process and one of the evangelists spreading the word about the benefits of 3D IM within the medical device industry.
An Introduction To 3D IM
The 3D IM process has a lot in common with traditional metal mold IM. To significantly oversimplify matters, 3D IM uses a 3D printer to create the A and B sides of a given mold out of plastic. The two parts of the mold are clamped together in standard IM equipment, and molten material is injected into the mold. Once the part is cooled, it is removed from the mold. Sounds pretty straightforward, right?
Not exactly, Darst told me. “Although 3D molding requires an injection molded press, it doesn’t necessarily follow all of the rules and techniques of traditional IM,” he says. “The molding conditions and settings are very different than they would be with metal tools. The process steps outside a lot of what you know about molding.”
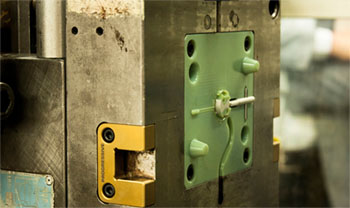
The novel 3D IM process allows 3D-printed molds to withstand the heat and pressure of injection molding machines.
The biggest difference is the heat profiles of the molds themselves. Molds made out of aluminum or steel can withstand extremely high temperatures and thus can handle materials with extremely high melting points. Many of the thermoplastics and other materials being used in today’s medical devices have melting temperatures of 500 degrees F or higher. 3D IM molds, however, are made out of a two-part plastic material called Digital ABS — originally developed by the Israeli 3D printer manufacturer Objet, now part of Stratasys — which will break down at constant high temperatures.
To circumnavigate this issue, 3D IM puts its unique spin on the standard IM process. The time between injection cycles is lengthened by up to a minute, to allow the surface of the mold to cool down to its optimal operating temperature before more molten material is injected into it. Using this modified IM process, 3D printed molds can produce as many as 25 to 100 parts before degrading — a far cry from the durability of a metal mold but more than sufficient for rapid prototyping purposes.
Earlier this year, Worrell decided to implement 3D IM in its model shop, investing in an Objet500 Connex from Stratasys and an 85-ton injection molding press from Toshiba. For larger parts, Worrell works with Advanced Molding Technologies, a neighboring injection molding company with a 250-ton press. The two companies have partnered in the development of the 3D IM process for medical device applications.
Worrell and Advanced are now using 3D IM to produce molds and prototypes for medical device makers using a broad range of materials — including some with surprisingly high melting temperatures. For instance, Worrell has been able to run a polycarbonate that melts between 540 degrees and 590 degrees F.
In addition to polycarbonates, the firm has also successfully run acrylonitrile butadiene styrene (ABS), nylons, Delrin (acetal resin), thermoplastic elastomers (TPEs), polyethylenes, polypropylenes, and other commodity-type materials and resins through 3D IM molds.
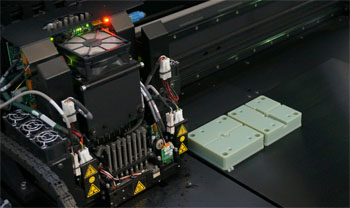
3D IM can reduce lead times by weeks — and costs by thousands — compared with traditional tooling.
“When we first heard about 3D-printed injection mold tooling with Stratasys Digital ABS material, we were apprehensive, especially due to claims made by competitors in the past,” said Matt Havekost of Advanced Technology Systems (ATS), the Stratasys reseller that helped Worrell select the right 3D printer for its business. “I will never forget the look on the face of the experienced injection molding press operator when the first part came off the 3D printed mold. That is something that sticks with me to this day as I talk with people about this exciting new application.”
Results: The Advantages Of 3D IM Over Conventional IM And 3D Printing
3D IM prototyping has two major benefits compared with traditional IM processes — namely cost and speed. “What makes 3D printed molds really appealing is you can mold out of Digital ABS material without having to make a large capital investment in aluminum or P20 steel tooling,” Darst told me. “And then you avoid the associated lead times that go with building metal molds.”
How much can 3D IM save you compared to metal molds? In most cases, Worrell has found that 3D IM molds cost 60 to 75 percent less than aluminum molds. Darst put it in practical terms: “If it is a $12,000 mold, you might be able to make it for $3,000 or $4,000, pending part geometry design, secondary options, functional requirements, and mold complexity.”
He pointed out an even more extreme example of the potential costs savings. “Stratasys is doing an enclosure for vein pumps in 3D IM that requires very complex tools. It is probably too expensive to do in aluminum tooling, because the prototype tool would be half of the cost of a production tool,” Darst said.
“At that point, you might as well build a production tool and then modify it, so you aren’t throwing away a hundred thousand dollars’ worth of work if mold changes become necessary.”
This highlights another plus of using 3D IM for prototyping — its design for manufacturing (DFM) capabilities. For instance, it can help identify potential problems with your design or tooling prior to building expensive production tools. “You can inexpensively work out the fits and the features that you need for assembly through 3D IM and mitigate some of the tooling-associated risk with creating a part,” he explained. “So when you do make the huge investment in tooling, you are pretty confident that your changes will be minimal and you aren’t throwing out an entire tool.”
As for the speed element, Darst says that some of the smaller 3D printed molds can be run in 5 hours, and his firm hasn’t encountered a mold yet that takes more than 24 hours to produce. That’s several weeks faster than for metal molds, and it doesn’t even take into account the possibility of mold rework.
“Metal tooling can take four to six weeks to get, and then you hope that your design is done, the molding conditions are right, and you can produce good parts. If not, you will need to make revisions. When all is said and done, you are at four to eight weeks before you get a good working part,” Darst said. “This 3D printing technology allows us to produce the mold in a day, and then we can be in the press the very next day. We can have parts within two to three days.”
MedTG, a medical device startup developing specialized catheter devices and associated technologies, recently partnered with Worrell on the design and engineering of a dual-flow needleless blood collection system that decreases the need for multiple injections. By using 3D IM to generate the prototypes, they were able to reduce costs by 70 percent and cut times by 95 percent compared with traditional tooling.
Compared with straight 3D-printed prototyping, 3D IM’s big advantage is the aforementioned ability to fabricate parts in parent materials, which helps streamline device validation and verification. Often, 3D-printed materials lack the tensile strength or other important properties of final production materials, making them unsuitable for true functional testing. Since 3D IM allows the use of many parent materials, prototypes fashioned this way are ready-made to be tested and submitted to the FDA. This adds to 3D IM’s lead in the speed department.
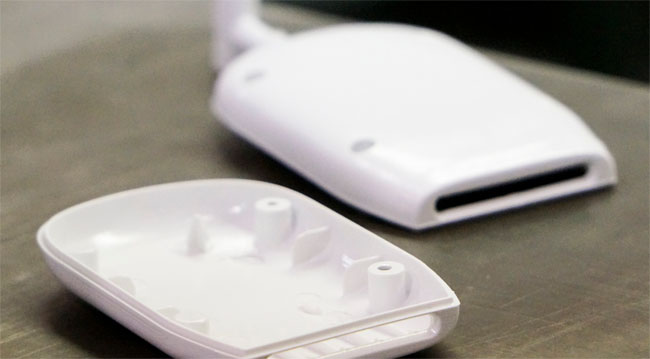
Worrell made these prototype parts for OBMedical’s LaborView maternal fetal sensor prototype using 3D IM.
“Imagine sending a part file to a product development firm and having a cavity and core for a mold completed in 16 hours,” Steve Jenkins, business development manager at Advanced, explained. “And in a matter of 24 more hours, you have molded parts in medical-specified resins in hand.”
For example, Worrell used 3D IM in the industrial design and engineering of OBMedical Company’s LaborView, a maternal fetal sensor system that measures labor contractions along with the heart rates of both mother and baby. Generating 3D IM parts in the parent material enabled OBMedical to progress quickly through electrical safety testing and submit final reports for its FDA submission, putting it way ahead of schedule. “Using the 3D IM process allowed us to move into safety and mechanical testing for our LaborView pilot devices in order to file for FDA clearance much more quickly and less expensively than originally anticipated,” Weaver Gaines, president and CEO of OBMedical, said.
Addressing 3D IM’s Shortcomings
No, 3D IM is not the perfect prototyping process, and it is not appropriate for all situations. While the number of materials that can be molded using 3D IM is impressive and growing, the plastic molds still can’t touch metal molds when it comes to materials that require extremely high heat or pressure to process.
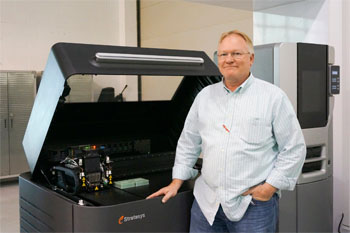
Worrell’s Dan Darst at the 3D printer his firm uses to produce injection molds.
In addition, the longevity of 3D IM tools is nowhere near that of molds made of steel or even aluminum. Best case scenario, your 3D IM mold holds up for 50 to 100 shots using a resin like polycarbonate, ABS, nylon, polypropylene, ethylene, or propylene, according to Darst. And some molds can only run a handful of shots. Mold durability is dependent not only on material selection but also on the complexity of geometry and the size of the part. Worrell tells clients to expect runs of 10 to 25 parts out of a 3D IM mold.
On the other hand, the fact that 3D IM molds are so cheap and quick to produce more than offsets their longevity limitations. “If your mold starts to break down or delaminate after 25 parts, the next day you could have another mold and run another 25,” Darst said. If you don’t want to wait a day to get a replacement mold, you can always print two of them at the same time.
Another area where 3D IM is imperfect (literally) is in surface finish. Since it is a layered process, 3D printing leaves small steps on the surface — it is not completely smooth or polished. And 3D IM can only deliver tolerances down to 2- to 4-thousandths of an inch, so extremely high-tolerance fits can also be an issue. However, Worrell and others have developed strategies to address these challenges.
One way to improve both surface finish and tolerance of 3D IM parts is by inserting aluminum inserts or pins into the mold before injection and then picking them out once the parts are molded. For example, Worrell worked on a valve design with small, thin fluid channels that ran through the main body housing and narrowed along the way. By using an insert in the 3D IM mold, the designers managed to achieve the tight tolerance and smooth surface required of the part.
“3D IM molds are very versatile. You can do insert molding, undercuts, threaded inserts, overmolding, and living hinges,” Darst stated. “You can do a lot of things you would traditionally do in an aluminum tool.”
What Lies Ahead For 3D IM
While there is certainly room for improvement, 3D injection molding is already being used successfully to produce functional medical device prototypes — and interest in the process is growing. “There is a very strong interest in 3D IM with everybody that we talk to, whether it’s a small startup company or a larger OEM,” Darst reported. “There is a great deal of attention being paid to this.”
Companies like Worrell, Stratasys, Advanced, and ATS are striving — often in collaboration — to improve and expand the 3D IM prototyping process. For example, Worrell is exploring the use of 3D-printed molds for use in blow molding, another area currently burdened by expensive tooling. Eventually, Darst expects to see 3D-printed molds move beyond prototyping and into short-run manufacturing.
In the meantime, proponents of 3D IM will continue to share its benefits and success stories with the medical device industry. “We are hopeful that this process is changing the way device makers look at innovation,” Darst said, “and we think it will fundamentally change the way medical devices are designed and developed.”