Can Aptamers In Wearable Sensors Mean A Revolution In Healthcare?
By Justin Gooding, Ph.D., The University of New South Wales and Nutromics Pty Ltd.
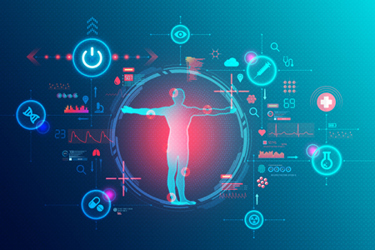
How can there be a revolution in wearable sensors, I hear you ask, when the field still seems so nascent?
Well, that is not entirely true because devices such as radiation monitors have been with us for decades. But like you, I was thinking about the smart watches by companies such as Apple, Garmin, Fitbit, and Suunto, to name just a few. These are watches with accelerometers, light emitting diodes, and pressure sensors that give us information about our activity, our heart rate, and our sleep. Much of this information can act as a surrogate for health information. Being a lover of data, I, for example, use my resting heart rates as a surrogate for when I am unwell or run down. I use the word “surrogate” here as these sensors all measure physical parameters, but your health and well-being status revolves around biochemical parameters. So, we use physical parameters to infer something about our biochemical status. This is a justifiable approach, especially as these physical sensors are capable of continuously acquiring information in real time over months or years without a deterioration in performance, batteries permitting, of course.
If it is viable to record physical surrogates as measures of how well you are, it is the way to go. However, physical information is not always a good surrogate of biochemical information. Or rather, the link is not well understood. There are times when we need to directly measure biochemical markers, and there are times when continuously monitoring biochemical markers can have important health consequences. So, the revolution I am referring to is a revolution in wearable sensors that monitor biochemical markers directly. This is something sensor scientists have dreamed about doing ever since the first biosensor was developed: the glucose meter, by Clark and Lyon in 1962.
Current Uses Of Biosensors
A biosensor is a sensor that uses a biological molecule, such as an enzyme or an antibody, to selectively detect a biomarker of interest directly in biological fluids. So, wearable biosensors can measure biochemical markers from blood, sweat, or interstitial fluid in a minimally invasive way. Some of you might say, but we already have these! And you would be right. We have continuous glucose meters marketed by diagnostics companies such as Dexcom, Medtronic, and Abbott. These devices show the power of continuous monitoring of biochemical markers, allowing people with diabetes to lead lives similar to those without diabetes. That has been an amazing advancement in healthcare. However, glucose sensors are a class of biosensors that use enzymes to selectively detect the targets of interest directly within a biological milieu. These are referred to as catalytic biosensors because enzymes are biological catalysts. The number of biomarkers that we might want to detect with catalytic biosensors is quite small, restricted to a few metabolites and other bioactive molecules.
There is a whole other class of biosensors that can detect biomarkers ranging from metabolites to drugs, proteins, and even viruses. This class of biosensors is referred to as affinity biosensors as it uses biological molecules like antibodies and DNA that have a high affinity for the biomarker of interest and bind them strongly. Chances are you have used one of these.
The rapid antigen tests and lateral flow devices used to detect COVID-19 and pregnancy tests are examples of affinity biosensors. As you know, you use these tests once and then throw them away. One of the reasons for this is that to achieve their selectivity for the biomarker of interest, the high binding affinity means that biomarkers bind and then do not let go from the affinity molecule. Hence, reversible binding to allow continuous monitoring is not an option.
Are Reversible Biosensors The Foundation For Personalized Care?
For decades, biosensor researchers dreamed about having affinity biosensors that were reversible, but most of us thought this was not possible. Then came one of the most incredible experiments reported in my career. Kevin Plaxco, a biological physicist from the University of California, Santa Barbara, published a paper on continuously monitoring drugs inside a living animal model in real time.1 I recall sitting in the audience at a conference in Sweden in 2015 when he spoke on this work, and I was stunned.
The key to achieving what I once thought was impossible was a new class of affinity biomolecule called an aptamer. Aptamers are sequences of DNA that do not bind to other nucleic acids but rather bind to small molecules and proteins like antibodies. By changing the sequences of bases in the DNA strands, you can change what the aptamer binds to and how strongly it binds. When the aptamers bind to the target, they change shape. Plaxco and his team took this feature and paired it with another they had pioneered. If a DNA sequence had an electrochemically active molecule on its end, then changes in the conformation of the DNA molecule attached to an electrode surface would give a change in electrochemical signal. The team called these new sensors electrochemical aptamer-based sensors, or EAB sensors for short. Even in that first paper, they demonstrated the capability for four drugs, not just one. In a few years, more than 20 drugs and metabolites have been monitored in living animals. The EAB sensor idea is general for many biomarkers; that is, the same basic technology can be used for many different molecules of interest.
So, why am I so excited about this development? It’s because it could revolutionize how we do healthcare. It takes us toward our 60-year-old dream of being able to monitor molecules inside the body and act instantly. Think about drug treatment. If an EAB sensor was applied to a patient, a clinician could monitor how much of a drug is in the patient’s body and personalize the dose to maximize the drug’s efficacy and minimize its toxicity. But, of course, there are lots of great ideas in scientific literature that we never see in our hospitals.
One example of a company aiming to bring this technology to market and approaching human studies is Nutromics. The startup is developing a patch that sits on a patient’s skin with tiny microneedles that sample the interstitial fluid. The EAB sensors are on the tips of the microneedles, which also serve as electrodes. The microneedle patch is pressed onto the skin, and the needles penetrate through the skin and measure the level of a drug in the interstitial fluid, just below the skin. Application of the patch is simple and painless. It is designed to sit on the skin for 24 hours, monitoring the level of a drug given to a patient in real time and telling the physician exactly how much more dose is required. Thus, it personalizes the treatment.
The first product Nutromics aims to develop is for the therapeutic drug monitoring of the antibiotic vancomycin, which has a very narrow therapeutic window, and the toxicity effects can be lifelong kidney issues or even death. The same technology could be used for other drugs, metabolites, and hormones. A patch could have several biosensors for several biomarkers, all on the same patch. Nutromics is not the only company exploring aptamer technology for detecting biomarkers. There are others in both Australia and the U.S., for example. There are also other companies using microneedles for sensing.
But what about protein biomarkers? Can we monitor those reversibly? Well, this is a much harder challenge, but one I am confident can be solved. Shana Kelley of Northwestern University, for example, has developed a molecular pendulum approach that was reported to be able to detect the cardiac marker troponin reversibly2 in Nature Chemistry in 2021. This suggests that this enduring challenge is also close to being solved.
Conclusion
If one starts to imagine a little, the healthcare revolution I mentioned earlier will come. Not only could a person’s care be personalized to maximize their treatment, but outpatients could be monitored after they leave the hospital to ensure their recovery is going well. If something goes wrong, they could quickly be alerted and brought back for further treatment.
This could enable decentralization of some pathology laboratory services and/or even pathology services based around much more accessible rapid diagnostics. Maybe we could prevent people from ending up ill by monitoring markers that are related to known causes of illness. Cortisol, for example, is a common stress biomarker, and chronic stress is thought to lead to a large number of illnesses. If a person could monitor their own stress levels or metabolites that show they are maintaining a good health status, perhaps some chronic conditions could be prevented. As I said, one can let one’s imagination run a little, but the ability to dream of a more personalized, less centralized, more home-focused care regime comes from a revolutionary experiment that shows us what might be possible when brave entrepreneurs are willing to shoot for that dream.
About The Author:
Justin Gooding, Ph.D., is a Scientia Professor at The University of New South Wales and is also chief scientific officer at Nutromics Pty Ltd., based in Melbourne. He has published more than 460 research papers and authored 14 patents. Gooding is known internationally for his work on surface modification, biosensors, developing functional nanomaterials, cell-based diagnostic devices, and electroanalysis.