Control and monitoring of bioburden in biotech and pharmaceutical cleanrooms
By Raj Jaisinghani, Technovation Systems, Inc.
Greg Smith, Encelle Corp
Gerald Macedo, MedPharmex
Introduction
This paper describes results of monitoring biotech cleanrooms and a pharmaceutical cleanroom equipped with an Electrically Enhanced Filtration (EEF) system that significantly reduces airborne bioburden in cleanrooms. The EEF HEPA system traps and kills bacteria and also improves the filtration performance of a filter media by two to three orders of magnitude. In laboratory tests the EEF technology has been shown to kill Staphylococcus epidermidis and Escherichia coli. These field test results support laboratory testing and show that basically there is no airborne bioburden in both a Class 10 room, with terminal HEPA in addition to the EEF, and in a Class 1000 room that utilizes only the EEF without any terminal HEPA filters. In the case of an old laboratory converted to a cleanroom, direct comparison of the EEF with respect to conventional HEPA fan filter units (FFUs) was possible. The results showed that at the same flow rate the EEF resulted in significantly lower bioburden as compared to the FFUs.
Background
The fundamental purpose of cleanrooms in the pharmaceutical, medical device, biotechnology and hospital applications is to control the amount of bioburden due to internal operations and due to transport from the air. From a particulate point of view, cleanrooms in these industries are classified and specified according to the same cleanroom standards (e.g. Federal Standard 209E) as in other industries. Often it is assumed that the particle (total) concentrations will generally correlate to concentration of viable microorganisms. This may not always be valid. Hence, the concentration of viable organisms is also directly measured - both at the work surfaces (or at the process) and in the air.
Cleanrooms in these industries must meet separate standards for bioburden. The FDA has specific requirements and guidelines for bioburden for various pharmaceutical operations and processes. Similarly, the USP and the European Union's GMP guidelines give specific recommended limits for microbial contamination for each class of room. A cleanroom that meets the particle concentration requirements, but does not result in the desired level of bioburden, will clearly be inadequate.
One of the main obstacles in achieving the required bioburden levels, is that the measurement of bioburden is time consuming. Typically, bioburden measurement involves sampling, incubation and counting of colonies. This is a time consuming process and thus "real" time monitoring is not possible. Thus, it is not always possible to relate higher incidents of bioburden to operating Recently, however, UV fluorescence (cf. Seaver and Eversole [1] Pinnick et al. [2]) technology has made it possible to achieve "real time" monitoring of particles of biological origin. This technology will find increasing use in the real time monitoring of air in hospitals, clean rooms and military nuclear, biological and chemical (NBC) warfare protection systems - as a real time supplement to the standard methods of determining bioburden. As this happens more attention will be focused on clean room contamination control systems - currently mainly mechanical filtration.
One problem with mechanical filters is that under certain conditions common bacteria caught on the filter can start growing on the filters, grow through the media and start shedding into the room [3,4,5]. The well known case of the Legionnaire's outbreak at the veterans convention in Philadelphia has been attributed to this phenomena. In that case the filters were supposedly in a wet state. Generally, it is accepted that bacteria is difficult to grow on clean glass fiber filter media, used in HEPA filters, under normal humidity conditions. However, since the function of these filters is to capture all particulate contamination, filters eventually get dirty. The experiments conducted by Jaisinghani et al. [6] show that very little contaminant is needed for growth of Staphylococcus epidermidis and Escherichia coli on HEPA glass filters. In their experiments Jaisinghani et al. [6] found that very little of the applied E. coli survived on the clean glass filter after four hours of air flow, keeping in mind that E. coli is not a hardy organism. Next about 1 gm of colloidal kaolin was added to the E. coli solution that was to be aerosolized. This time the recovery of E. coli was about 104 - 105 CFU/square inch of the filter media. Similar tests with S. epidermidis recovered a little more S. epidermidis than with E. coli even without the colloidal kaolin, due to the hardier nature of S. epidermidis. With 1 gm of colloidal kaolin in the 25 ml S. epidermidis solution (in tryptic soy broth) the recovery of S. epidermidis was about 105 - 106 CFU/square inch of filter media. Seven hours of continous air-flow tests (following the aerosol) did not result in any significant reduction in bacteria recovery. This result suggests that, even in normal environments, bacteria can survive or grow on the filters. As the trend towards using HEPA clean room filters for longer periods continues, the possibility of bacterial growth on the filter, and thus the rise in the airborne bioburden, also increases.
This paper discusses the bactericidal properties on an ionizing electrically enhanced filtration technology, and how this affects bioburden in cleanrooms.
EEF Technology
Jaisinghani [5] has played a significant role in the development and commercialization of EEF technology. The most recent version (see Figure 1) of this technology [5] maintains the filter under an ionizing (as opposed to a simple electrostatic field) field. Another higher intensity ionizing field charges incoming particles, stabilizes the electrical fields and increases the safety and reliability by ensuring that no spark over can occur towards the filter. This method provides two fundamental benefits:
- Bacteria are killed as they pass through a first high intensity ionizing field and then killed as they are subjected to continuous ionizing radiation when they are trapped on the filter. This inhibits growth of bacteria on the filter.
- The same ionizing fields enable penetration reduction by about two to three orders of magnitude.
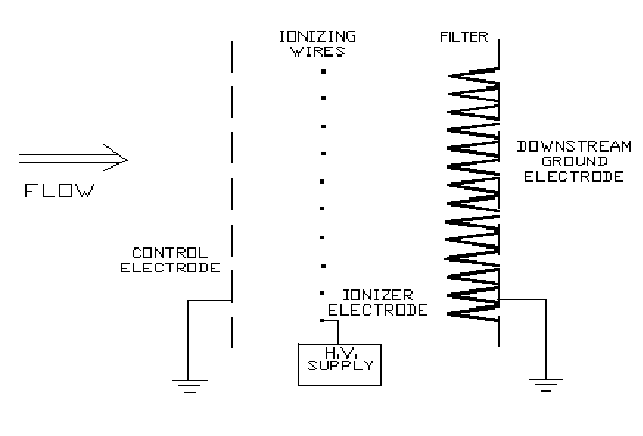
Since the cost of the additional electrical components is partially offset by the increase in filtration performance (either higher flow at the same pressure drop and filtration efficiency or lower pressure drop at the same flow and efficiency, as compared to mechanical filtration of the same size) this is a highly cost effective method for the control of bioburden.
Laboratory Evaluation of the EEF
Jaisinghani et al. [6] have demonstrated the bactericidal properties of the EEF under laboratory conditions. This study, conducted at Virginia Polytechnic Institute, is summarized in this section.
Experimental methods
S. epidermidis was grown in Tryptic Soy Broth (TSB) to a concentration of 3x109 colony forming units (CFU)/ml. The culture was lyophilized in Wheaton vials in 5 ml aliquots - 1.5x1010 CFU per vial. All vials were stored in a desiccator at 4-6 C.
Pleated 15.24cmx15.24cmx5cm (6"x6"x2") deep filters were first coated with colloidal kaolin and TSB using an Aztek airbrush. The airbrush cup was filled with 1g kaolin suspended in 25ml TSB and sprayed onto a filter inside a laminar flow hood and allowed to air dry before being used. The pleated filters were placed in a miniature version of the EEF. One vial of lyophilized S. epidermidis was resuspended with 1 ml of sterile distilled water. A small aliquot of this suspension was serially diluted ten-fold to 10-8 and plated on Columbia Blood Agar (CBA) plates to confirm the initial viable concentration of bacteria. The rehydrated culture was then sprayed onto the filter using a Meinhard nebulizer, which was placed 8 inches from the center of the filter.
A control assay was performed to determine the amount of viable S.epidermidis on the filter, without application of high voltage. The bacteria were sprayed onto the filter as previously described, and the temperature and humidity were monitored every 15 minutes for four hours or seven hours during which the air flow was on. The relative humidity was held between 45-55% using a Kaz steam vaporizer. At the end of each control run three pieces of the filter were extracted using a sterilized scalpel and forceps. The pieces of filter were approximately 1 square inch on the face of the filter, which when unfolded measured approximately 28 square inches of filter material. Filter pieces were removed from the center of the filter, directly above the center, and directly below the center.
The samples were cut into small pieces and placed into 10 ml of sterile phosphate buffered saline (PBS) pH 7.4 in a Nasco Whirl-Pak bag. The bags were then processed in a Tek Mar Stomacher Lab-Blender 80 for one minute. Each sample was then serially diluted ten-fold to 10-2, 10-3, and 10-4, then spread on CBA plates to determine the number of viable bacteria per sample filter piece.
Similar tests were then conducted by applying high voltage to the EEF. In addition to monitoring the temperature and humidity, the current was also monitored at fifteen minute intervals during the 4 or 7 hour period of air flow with the applied high voltage on.
Results and discussion
The results are summarized in Table I. In the absence of any voltage applied to the EEF unit (i.e. control tests), viable bacteria were recovered from 1 sq. in of filter in the range of 1 x 105 CFU to 2 x 106 CFU. Counts greater than about 3 x 106 CFU were too crowded to be accurately counted and were considered to be TNTC (too numerous to count). When high voltage was applied for 4 hours the majority of the bacteria were killed. The kill rate increased with increased voltage or with the first applied field strength (applied voltage divided by the distance of the ionizer wires from the control ground electrode - see Figure 1), V/d1. At a field strength (V/d1) of 4.2 kV/cm, there was no growth after 24 hours of incubation. After 48 hours, there was either no growth or small (in size and in number) colonies grown. These small colonies were identified as S. epidermidis, and were identical in biochemical profile as the isolate used in the tests. It was concluded that 4 hours at 4.2 kV/cm (V/d1) did not completely kill the S. epidermidis. If the bacteria were not all killed, some of them were damaged sufficiently so that no growth or very limited growth could occur after 24 hours incubation. When the ionizing time was increased to 7 hours, over 99% of the bacteria (as compared to the control) were killed.
When the applied field strength, V/d1, was increased to 4.5 kV/cm or higher, no growth occurred on any of the filter pieces except for one experiment. This exception may have occurred because the starting dose of bacteria for this experiment was 3 times higher than for the control and up to 10 times higher than for any other experiment. Nonetheless, there were still 3-4 logs of killing using an applied field strength, V/d1 of 4.5 kV/cm or higher, as compared to the control experiments. It should be noted that, in practice, bacteria caught on the filter are held within the ionizing field for an almost infinite amount of time, thus receiving an almost infinite radiation dosage. Hence, in practice, the killing efficiency should be higher even at lower field strengths. Similar results were obtained using E. Coli in a previous study conducted with the EEF at the University of Wisconsin.
For more information: Rajan Jaisinghani, Technovation, 13511 East Boundary Rd., Suites D/E, Midlothian, VA 23112-3941. Tel: 804-744-0604. Fax: 804-744-0677.