Ingestible Diagnostics: Technical Challenges, Regulatory Considerations, and Reimbursement
By Yoav Kimchy, Ph.D., Check-Cap
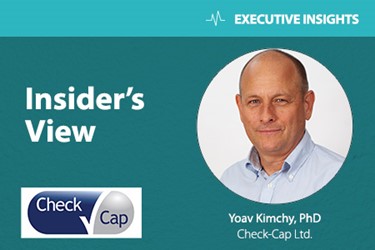
A number of ingestible technologies have been developed for the diagnosis of gastrointestinal (GI) diseases, including the use of conventional tools, such as endoscopy, radiological imaging, or biochemical tests[i],[ii].
Ingestible diagnostics are particularly useful for the exploration of the small bowelii, since gastroscopy can only reach a limited area of the small intestine and alternative methods with further reach, such as balloon enteroscopy[iii], require specific expertise and infrastructure. Ingestible devices have also been developed for exploration of the large bowel in search of polyps and other abnormalities, such as bleeds. A major clinical advantage of ingestible diagnostics relates to the friendliness of procedures, since patients can avoid invasive tests and be scanned while continuing their daily routines.
Innovation in material science, electrical engineering, computational tools, and data science, as well as widespread physician acceptance of currently approved technology, has triggered the development of ingestible diagnostic technology with expanded applications and, in some cases, improved diagnostic outcomes [iv]. However, several factors must be considered to ensure the development of ingestible diagnostic devices that are clinically valuable, safe, and cost-effective.
Technical Challenges in Ingestible Dx Development
Challenges associated with the development of ingestible diagnostic technology — that influence safety and device performance — include size, tracking and communication system type, battery life, and sensor type.
Size — Ingestible electronics require numerous synergistic components, including sensors, connectivity systems, and powering mechanisms[iv]. These components all need to fit into a capsule small enough to swallow and travel safely through the GI tract. While larger capsules might allow for more complex functionalities, device size is directly associated with capsule retention[v], which is linked to GI obstruction. As such, device size is a crucial design consideration.
Most current complex ingestible diagnostic capsules in commercialization or development fall within the centimeter size scale due to their range of batteries, communication systems, and complex electronics. Retention of endoscopy capsules several centimeters in length was reported, in one study, in 1.4 percent of a population of 1,000 subjects suspected of bowel disease[v]. It is recommended that capsule retention rates from non-deformable, pill-shaped devices with known safety profiles[iv] be explored as a reference point for safe designs relevant to the design of ingestible electronics.
Future developments to reduce size include the exploration of different geometries, as well as the use of edible or biodegradable components with the ability to disintegrate. Smaller powering systems, enabled through the development of alternative energy delivery approaches, also are being explored.
Tracking and Communication Systems — In addition to a functional capsule, ingestible diagnostic technologies need to fully integrate with a communication and tracking system. This system receives and stores information from the capsule that is later downloaded into a computer workstation, enabling GI specialists to analyze the information. The tracking system usually comprises a number of sensing pads that attach to the patient, as well as a data recorder and a battery pack[vi].
Data from the capsule must be wirelessly communicated in real time to the tracking system so physicians needn’t retrieve the capsule once excreted. Most devices use far field radio frequency as the technology for wireless communication[iv]. Far field radio frequency allows for communication, but with high signal attenuation (about 2.5db/cm).
Since capsule size constraints must be considered, other transmission methods have been used, including ultrasound-mediated communication or body coupling, as well as electric and magnetic near-field systems, which were used in early capsule designs. The disadvantage of electric and magnetic near-field systems is that the signal is available only along a limited number of axes, which links data reception to capsule orientation. One solution is to add multiple transmitters and receivers to gather signals from multiple orientation axes, but these transmission methods have limited communication range.
As such, ingestible device designers must balance maximization of signal transmission efficiency against capsule size. Some options being considered include the use of biocompatible and transient antennas that only expand when needed, or that can degrade to avoid obstruction[iv].
Battery Life — Size is not the only consideration for powering systems within capsule devices. Batteries also have to produce long enough for the system to collect all required measurements.
This is a small challenge for those devices targeting the upper GI tract — such as the esophagus, stomach, or even the small bowel — since battery lives of 20 minutes for the esophagus or 8-12 hours for the small bowel are generally acceptable[vi]. However, devices targeting the large bowel, including colon and rectum, need battery lives of 100 hours or longer to accommodate patients with slow transit.
Sensor Type and Endurance — While a number of exciting indications and opportunities are being explored for ingestible diagnostics, each must develop sensors that can withstand the harsh and caustic environment in the GI tract. Further consideration should be given to the potential need for bowel preparation ahead of procedures using ingestible diagnostics.
For instance, current devices that seek structural abnormalities include camera systems that require patients to undergo some type of fasting and bowel preparation ahead of procedures to ensure optimal visibility. While visibility of the upper GI tract (e.g., esophagus and small bowel) typically is easily ensured with light fasting and bowel preparation protocols, proper cleansing of the colon requires intense bowel preparation protocols not tolerated or accepted by many patients.
As with any medical device, patient compliance or acceptance of procedures must be considered when developing ingestible diagnostics. For instance, in the case of colorectal cancer screening, almost half of the population in the U.S. avoids current screening methods[vii] due to the invasiveness of the colonoscopy procedure and bowel preparation regimes associated with camera-based methods.
One consideration already in development is the creation of ingestible technology for prep-free screening[viii]. Instead of cameras, this technology uses ultra-low doses of x-rays to determine distances between the capsule and the inner walls of the colon and rectum. Ultimately the technology generates a 3D reconstruction of the GI tract, allowing for detection of structural abnormalities, such as precancerous polyps.
Working Station — The third component of ingestible diagnostic technology is a working station, where data received by the tracking system is downloaded for further analysis by a technician, followed by a GI specialist signoff. Alongside a functional capsule and tracking system, appropriate analytic software and algorithms are crucial to both accurate and patient-friendly analysis of data collected.
Current devices require patients to go back to the clinic so data from the tracking systems can be downloaded directly. In hopes of expediting and facilitating diagnosis, device developers have considered adding features that enable direct transmission of data to patient cell phones and virtual reading centers.
Regulatory and Reimbursement Considerations
No matter how good a device’s clinical efficacy profile, it must demonstrate real value: here, meaning a similar or lower cost and improved outcomes. Obtaining approval for commercialization is only the first step. The developer then must ensure the device fits into the reimbursement and insurance landscape so healthcare providers can use and recommend the technology to patients.
Conversations around reimbursement should be initiated as early as possible in the development process so the added value of the device can be evaluated and demonstrated. For instance, traditional endoscopy continues to be the gold standard and the most cost-effective solution for the diagnosis of many conditions[ix], such as colorectal cancer screening. But ingestible diagnostics (particularly prep-free options) have the potential to increase screening adherence and as such, this additional value should be taken into consideration when discussing reimbursement options.
Similarly, device manufacturing is costly, requiring the invention and integration of new computational technologies and software. Manufacturing partners typically consider their investments in manufacturing lines while ensuring a return value[x], which is strongly linked to reimbursement options.
Unfortunately, medical device reimbursement policy can hinder healthcare providers’ and patients’ access to innovative medical technologies, including ingestible diagnostic devices [x]. It can impede medical device companies from advancing innovative solutions, even if new technologies could lower costs and improve health outcomes in the long term. Demonstrating clinical potential early has its challenges — including the submission of clinical evidence through the development of clinical trials, which can place significant costs on emerging medical technology companies.
Companies seeking FDA approval for devices can potentially leverage data from clinical trials in the EU and elsewhere to start conversations about reimbursement coverage before initiating clinical trials in the U.S. Indeed, international agencies like the World Health Organization (WHO)[xi] and the International Organization for Standardization (ISO)[xii] have developed initiatives over the years to advance global harmonization of medical device regulations. This includes publication of a set of voluntary international standards — relating to product development, manufacture, quality assurance, and control — to provide various governments with a technical base for health, safety, and environmental requirements.
Finally, it is important to initiate conversations with manufacturing partners to ensure their capability to scale up production and distribution of your devices while maintaining adherence to regulatory and safety standards across global markets.
Conclusion
The field of capsule diagnostics is quickly advancing and has the potential to revolutionize the GI diagnostics field[xiii]. Further enhancements in technology and computation, together with the development of innovative materials, will allow companies to continue to improve these devices so they can become the standard of care in the future.
In addition to monitoring structural changes, ingestible diagnostic devices are being considered for microbiome sampling, gas and luminal content monitoring, motility and functional studies, drug delivery, and monitoring of medication adherenceiv].
We should expect innovation and great advancements in this field in the coming years that will provide us with an unprecedented understanding of GI health and diseases.
About The Author
Yoav Kimchy, Ph.D., has more than 20 years’ experience in developing and managing medical device companies. He founded Check-Cap in 2005 and has served as the chief technology officer since 2009. Before founding Check-Cap, Dr. Kimchy served as an algorithm specialist and technical project leader at a government contract company; director of cardiovascular research at a biomedical company; and VP of R&D at a medical device company focused on nuclear molecular imaging technology. Dr. Kimchy received his doctorate from the Technion Israel Institute of Technology.
[i] Ang D, Fock KM, Law NM, Ang TL. Current status of functional gastrointestinal evaluation in clinical practice. Singapore Med J. 2015; 56:69–80
[ii] Nguyen VX, Le Nguyen VT, Nguyen CC. Appropriate use of endoscopy in the diagnosis and treatment of gastrointestinal diseases: up-to-date indications for primary care providers. Int J Gen Med. 2010; 3:345–357.
[iii] Xin L, Liao Z, Jiang YP, Li Z S. Indications, detectability, positive findings, total enteroscopy, and complications of diagnostic double-balloon endoscopy: a systematic review of data over the first decade of use. Gastrointest. Endosc.2011; 74, 563–570.
[iv] Steiger C, Abramson A, Nadeau, P et al. Ingestible electronics for diagnostics and therapy. Nat Rev Mater. 2019; 4, 83–98.
[v] Li F, Gurudu SR, De Petris G, et al. Retention of the capsule endoscope: a single-center experience of 1000 capsule endoscopy procedures. Gastrointest. Endosc. 2008; 68, 174–180.
[vi] Wang A, Banerjee S, Barth BA, et al. Wireless capsule endoscopy. Gastrointestinal Endoscopy. 2013; 78, 805 - 815
[vii] Colorectal Cancer Facts & Figures. American Cancer Society. 2017-2019
[viii] Gluck N, Shpak B, Brun R, et al. A novel prepless X-ray imaging capsule for colon cancer screening. Gut. 2016; 65, 371–373.
[ix] Hassan C, Zullo A, Winn S, Morini S. Cost-effectiveness of capsule endoscopy in screening for colorectal cancer. Endoscopy. 2008; 40, 414–421.
[x] The Impact of Medical Device Reimbursement on Cost, Innovation and Health Outcomes. 2014. 13-000949. GE Healthcare publication: http://files.publicaffairs.geblogs.com/files/2014/08/The-Impact-of-Medical-Device-Reimbursement-on-Cost-Innovation-and-Health-Outcomes.pdf
[xi] Medical device regulations: global overview and guiding principles. World Health Organization. Geneva. ISBN 92 4 154618 2
[xii] ISO 13485, Medical devices – Quality management systems – Requirements for regulatory purposes.
[xiii] Goenka MK, Majumder S, Goenka U. Capsule endoscopy: present status and future expectation. World J. Gastroenterol. 2014; 20, 10024–10037.