Leveraging Electronics Manufacturing To Build Living Tissue
By Chuck Seegert, Ph.D.
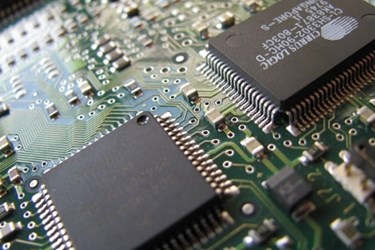
A new device that incorporates concepts from electronics manufacturing has been used to build tissue-like constructs. From cell-laden, tissue-engineered subunits, larger living aggregates have been assembled in a manner that resembles the pick-and-place automation used in electronics. Current work has led to assemblies with cell densities similar to natural tissues, and making organs could be the next step.
Creating replacement organs on-demand has been a long time goal of tissue engineers, but it still hasn’t been achieved. Many attempts at printing living tissues have led to some interesting results, but creating constructs with similar cell densities to those seen in natural tissues has been a challenge. Tissues are complex assemblies that exist on large scales when compared to the cells that compose them. These complexities may be similar in some ways to computer circuit boards, which are relatively large compared to their individual components.
Manufacturing large volumes of electronics has traditionally depended on robotic assembly methods that pick and place parts in a precise fashion. This automated manufacturing system takes many small components — like resistors and chips — and places them on a board to create a relatively large and complex product. Using similar principles, researchers at Brown University have developed a pick-and-place system for living, tissue-like assemblies, according to a recent press release. Each subunit is a scaffold with cells that are grown on it in culture.
The device is called the “Bio-Pick, Place and Perfuse (Bio-P3), according to a study published by the team in Tissue Engineering Part C: Methods. Once each subunit scaffold is cultured, the pick-and-place device takes them and precisely assembles them into a larger organized structure. The device provides nutrients by perfusing the subunits during assembly and after the larger tissue-like construct is created. In the study, scaffold subunits were created in honeycomb, toroid, and spheroid geometries, which were manipulated by a probe that used a slight negative pressure to grip them. This transport and release of the scaffolds has little to no effect on cell viability.
Unlike bioprinting methods that deposit small droplets one at a time, the new approach uses much larger subunit scaffolds that contain up to 250,000 cells each, according to the press release. Combining four of these honeycomb scaffolds together led to a 2-millimeter thick proof-of-concept assembly consisting of about one million cells. Furthermore, these scaffold subunits began fusing together after a short time in the nourishing environment, forming a cohesive large-scale structure.
The team’s existing design manipulates the scaffold subunits manually, according to the press release. With a recently received $1.4 million grant from the National Science Foundation, however, the team plans to make major improvements to the technology that may include automation.
Assembling 3D tissue-engineered implants is being intensely investigated by many research teams. For example, in a recent article on Med Device Online, a partnership between researchers at Yale University and a company specializing in 3D biology was described.