Medical Device Biocompatibility Testing: Best Practices & How To Minimize Risks
By Jason Song, SureMed Technologies
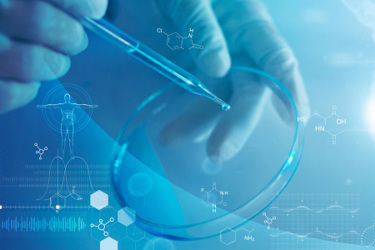
In Part 1 of this two-part series, I discussed strategies to ensure biocompatibility success from an organizational standpoint. In this second part, I will briefly discuss some of the ways to ensure biocompatibility study successes, as well as cross-leveraging or cross-product biocompatibility synergy. I will not go into detail on “nature of body contact and contact duration” of the medical device and associated biological safety endpoints as recommended by ISO 10993-1. The selection of the appropriate body contact category and contact is well defined in the ISO 10993-1 standards, as is the duration of contact with the body (A, B, or C).
To start, as in the standard’s name (Biological evaluation of medical devices – Part 1: Evaluation and testing within a risk management process), ISO 10993 operates within the risk management process, aligned with regulatory authorities’ risk-based approach to medical devices and pharmaceutical products.
In the updated version of the ISO 10993-1 standard, as well as the FDA guidance document, the use of chemical characterization as a tool to demonstrate biological safety is emphasized. This is a very powerful tool for biocompatibility planning, biological safety assessment, and cross-product leveraging.
One of the first steps in evaluating biological safety is understanding the material’s physical and chemical properties. Chemical characterization results are a key element to take into consideration when determining choice of test procedures. Table A.1, “Endpoints to be addressed in a biological risk assessment,” of ISO 10993-1 was updated to include physical and/or chemical information as prerequisite information needed for risk assessment.
The addition or emphasis of chemical characterization within biocompatibility is viewed by many experienced biocompatibility toxicologists as attention to an often-overlooked tool that has been heavily utilized in the past to provide biological safety assessments and justifications in lieu of testing, as allowed by current and previous versions of ISO 10993-1, the FDA guidance document, and the FDA’s 1995 memo #G95-1. In fact, the updated FDA guidance incorporates a decision tree that was built off the decision tree backbone from #G95-1. Chemical characterization is a long used but not well documented tool to help justify biological safety and determine atypical testing schemes to demonstrate a device meets biocompatibility endpoints. It has saved clients time, heartache, and cost as well as minimized animal testing. The updated ISO 10993-1 standard now clearly states how chemical characterization can be used to generate data to support biological safety assessment, demonstrate equivalence with a previously assessed medical device or material with established safety, and/or justify reduced testing.
While chemical characterization when executed correctly can generate compelling data to help justify or leverage prior data to minimize biocompatibility testing, it also should be noted that it can rarely be used as a stand-alone justification without support of additional data, be they reduced biocompatibility testing, epidemiology, or other data sources.
This brings me back to the importance of having a biocompatibility toxicologist experienced in both engineering and toxicology, as well as incorporating the biocompatibility toxicologist within the product development team from the onset. This is because to demonstrate equivalence, it is essential to demonstrate not just that the product’s materials are equivalent but also that the manufacturing process and functionality of the medical device will not result in the addition and/or creation of byproduct chemicals and/or residuals that may cause harm to the user or patient.
As an example, two products used in invasive surgical devices with the same material of construct may on the surface appear to be equivalent. But one may have a manufacturing step whereby the material is exposed to a chemical agent that results in surface material degradation, or the material may be exposed to an elevated manufacturing processing temperature whereby an unsafe byproduct is produced. These changes may render the materials not equivalent, and biocompatibility testing will result in one or more of the tests failing. As such, when assessing equivalence, a number of biological/physiological as well as mechanical, engineering, and process elements, such as the device’s mode of action, contact duration, and exposure to the environment, need to be considered and factored into the risk assessment and justification for leveraging. The environmental exposure we are talking about here goes beyond the physiological environment to include the surgical environment and other fluids the product may come into contact with. For example, a drug delivery catheter may have the same material of construct, manufacturing process, and chemical characteristics as a stent delivery catheter but depending on the formulation of the drug product the drug delivery catheter will be in contact with before, during, and after delivery, there might be a material incompatibility that results in biologically unsafe byproducts.
When assessing a manufacturing process for biocompatibility risks, it is essential to understand that contribution to such risks is not equal among all the steps. Steps early in the manufacturing process and steps prior to any wash and cleaning step pose a lower risk than subsequent manufacturing steps. Furthermore, the biocompatibility toxicologist should also understand the potential impact various sterilization methods have on materials and their impact on biocompatibility. Gamma irradiation and e-beam sterilization of incompatible materials such as certain elastomers and plastics may result in gradual material degradation that is not detected until later in the product’s shelf life and degradants found to pose a biological safety risk to end users and patients during biocompatibility testing. Similarly, gas sterilization such as ethylene oxide can leave residuals that are not safe when the out-gassing duration is too short or produce ethylene glycol if the product is not fully dry before sterilization.
The reality of product development is that not all processes or materials can be changed; some processes and materials are essential and cannot be substituted. When this happens, the biocompatibility toxicologists can assist the team in carrying out appropriate design of experiments (DOE) with the development engineer, using representative materials and appropriate characterization methods (i.e., chemical) to help establish parameters and process combinations that would render the final product safe. An example of this may be when a chemical must be used on a slightly incompatible material; in this case, the biocompatibility toxicologist and development engineer can devise appropriate DOEs whereby maximum exposure duration is established and appropriate subsequent neutralizing agents and/or wash steps used so the finished product is biocompatible. The selection of appropriate characterization methods, such as gas chromatography – mass spectrometry (GC-MS), inductively coupled plasma – optical emission spectrometry (ICP-OES), and Fourier transform infrared spectroscopy (FTIR) to name a few, is vitally important and can be the difference between successful DOE and inconclusive DOE. Here, the biocompatibility toxicologist not only can help identify the right characterization assays but also determine the right engineering parameters to test.
To reemphasize: Early involvement by a biocompatibility toxicologist can help de-risk a program from material selection to process development and assist in establishing appropriate DOE process parameters. Next, I’ll discuss how to minimize risks when performing biocompatibility testing.
How To Minimize Risks When Testing For Biocompatibility
First, biocompatibility testing should be done only on user and patient contact areas. Biocompatibility testing should not be performed on the whole device. Internal components have no business being tested unless the internal material can migrate to a surface a user or patient may contact, such as, for example, lubricants used within a device that both have a tendency to and can migrate out during use.
It should be noted that when performing biocompatibility testing, it is typical for the device to be disassembled or cut so that only the user- and patient-contacting materials are used for extraction. While samples will most likely be cut, the sample should be representative of the final product as manufactured using the final manufacturing process.
The user and the patient for a medical device may be more than one person, such as in the case of a drug delivery device for children, where the user may be the parent and the patient, the child. As such, it is OK for a medical device to have different surface contact classifications for different parts of the device. A part of the device may be classified as “intact skin” contacting while another part may be “blood path, indirect.” In such a case, the “intact skin” portion should be assessed based on its contact type and duration, while the “blood path, indirect” portion will be assessed appropriately for that classification and its contact duration. It should be noted that in the case of healthcare providers, where the user would be wearing gloves, the glove acts as a barrier and the glove contacting portion of the device will not need to undergo biocompatibility.
At this point, it should also be noted that the new ISO 10993-1 included a transitory-contacting classification. These are devices or parts of devices with a very brief/transitory contact with the body, such as lancets, hypodermic needles, and capillary tubes, that are used for less than 1 minute. In this case, those parts of the device would not require testing to address biocompatibility. But the caveat here is that if those devices’ elements or parts have coatings or lubricants that can be left on body tissue in the course of use, at minimum a biological safety assessment will need to be done assessing the residues.
While ISO 10991-1 Table A1, as well as the Biocompatibility Evaluation Endpoints table in the FDA guidance document, provides guidance on the minimum test categories to be performed, you need to select the right test and/or testing parameters. For example, there are a number of cytotoxicity test types that can be performed. By far, cytotoxicity is the most common biocompatibility test program that has experienced failing results. That’s because there are a number of different cytotoxicity tests that one can choose from and sometimes test labs recommend the most convenient test, as opposed to the right one for your product. Cytotoxicity tests to choose from include agar overlay, MEM elution, and direct contact, just to name a few. The selection of the appropriate cytotoxicity tests will depend on such things as your device’s user/patient contact surfaces. A product that is intended for intact surface contact, when subjected to an MEM elution run, has a high risk of failing cytotoxicity. Similarly, a laparoscopic surgical device may pass an agar overlay cytotoxicity test, but it would likely be called into question, as an agar overlay is intended for contact with intact skin and will not provide the appropriate cytotoxicity assessment on an invasive product.
In addition to selecting the right test, the right elution medium, temperature, and duration are just as important. While I can go in depth on physiology and selection of the right elution medium, in general the elution medium should match the body environment the device is intended to be used in.
Conclusion
Establishing a product development process in which a biocompatibility toxicologist is included early in the program to assist with material selection, process development, etc. from a biocompatibility/product biological safety standpoint will help ensure product development and biocompatibility success as well as avoid biocompatibility mediated redesigns or process changes. Having a biocompatibility toxicologist who has both engineering and toxicology experience will further help de-risk the program’s biocompatibility and allow for the development of strategic and streamlined biocompatibility approaches. Finally, when performing biocompatibility studies, attention should be paid to identification of the user and patient contact areas, selection of tests, and appropriate testing details.
If you would like to discuss this topic or have more specific questions related biocompatibility or biological safety (toxicology) assessment, please feel free to contact me directly. If you have any other topics or questions you would like to see covered or discussed, please drop a line in the comments section or reach out to me directly at jsong@suremedtech.com.
About The Author:
Jason Song, P.E., is chief technology officer of SureMed Technologies, Inc., a company he co-founded in 2018 that develops holistic novel technologies, products, and services that balance the needs of patients, industry, and the healthcare ecosystem. Previously, he held various technical and leadership positions at Amgen, Eli Lilly, GE Healthcare, Motorola, and Novo Nordisk in a range of areas, including injectable and inhaled drug delivery device development, fill and finish, packaging and assembly automation, biochips, battery development, and establishing new production sites. He holds BS and MS degrees in mechanical engineering, an MS in automation manufacturing, and an MBA.