Medical Device Biocompatibility – Toxicological / Biological Safety Assessments
By Michael Song, Ph.D., AstraZeneca
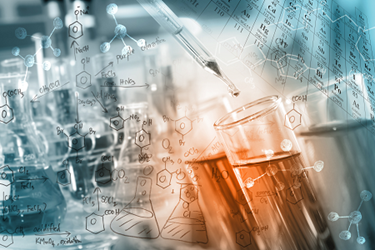
Whether you are working on 510(k)-exempt devices, 510(k) devices, or PMA devices, ISO 10993 biocompatibility is an essential element.
Given the variety of medical devices, ISO 10993 provides guidance on recommended biological endpoints to assess, based on how the medical device interacts with the patient. For example, the device may interact (“nature of body contact”) via surface contact mucosal, externally communicating blood path indirect, or implant. Duration of contact also must be considered in terms of contact duration A (<24 hours), B (beyond 24 hours; up to 30 days), or C (beyond 30 days).
Despite ISO 10993’s utility, there are always new technologies, devices types, and materials that may warrant additional considerations based on patient health risks — for example, 3D-printed medical devices or devices made out of novel nano materials. Device biocompatibility is a vast topic and, as such, we will only cover it here at high levels (please feel free to contact me with more specific questions).
This article focuses on how to handle situations where deviating from recommended testing may be desired, either due to timing or a desire to leverage prior biocompatibility and periphery data in support of a biological safety assessment. In these cases, a biological safety / toxicological assessment is warranted.
When and How: Toxicological / Biological Safety Assessment
This approach has become more popular among medical device companies seeking to minimize animal testing and maximize the use of existing data. For example, for a 510(k)-exempt, surface-contacting medical device (e.g., an ECG cable), the recommended tests are cytotoxicity, sensitization, and irritation. Sensitization and irritation are both animal tests. This is in line with the recent update of ISO 10993-1, where a noticeable a shift in preference toward leveraging prior and existing biocompatibility or material safety data can be clearly seen. This is, in part, an effort to reduce animal testing.
A toxicological / biological safety assessment, in essence, utilizes the risk-based approach ISO 10993-1 follows. In addition, the FDA guidance document on applying ISO 10993-1 emphasize the role risk assessment and management have in evaluating a medical device’s biocompatibility.
The updated ISO 10993-1 provides guidance and recommendations for users to assess a medical device’s materials of construct — as well as whether the material has a history of safe use in similar applications — as part of an initial assessment to determine which biocompatibility tests are still warranted. In fact, Table A.1 of ISO 10993-1 added a column for physical and/or chemical information to further encourage users to examine, initially, their device manufacturing process and materials of construct to determine any additional biocompatibility assessment necessary.
Related, both ISO 14971 and ISO 24971 refer to biocompatibility as an area where ISO 14971’s hazards and risk approach can be applied. ISO 24971 also notes how ISO 10993-1 aligns with ISO 14971’s risk-management approach. However, when performing a biological safety assessment under ISO 10993-1, one must consider additional contributing factors that may impact a medical device’s biocompatibility, from the manufacturing process and chemicals used to the device’s functionality.
The intent of biological safety / toxicological assessments is to indirectly determine whether a medical device is safe from a biocompatibility standpoint. As such, a number of data and data sources may be utilized, including, but not limited to:
- supplier data and/or raw material compliance data
- predicate device data
- periphery internal development work, such as cleaning validation.
Still, one should realize that there is no “magic bullet.” That is, it is rare to find a single data source that can provide all the necessary support to prove your device’s biocompatibility. Often, it takes a mixture of data to prove your device is biocompatible. In addition, data from those sources may not be a direct one-to-one translation to your device; as such, appropriate safety factors need to be added to utilize those data.
Consider a material widely used on consumer products, as well as medical devices. When evaluating such a material and its biocompatibility in your device, it is important to consider the current medical device and its mode of action or use situation. For example, the material may have been safely used on an surgical staple, but if your device is a directed energy device (e.g., a bipolar laparoscopic sealer / divider), the material’s effectiveness on a surgical staple may not translate to your device, as it has not been near or in direct contact with the heat-generating element of a bipolar electric sealer / divider. In such a case, additional data will be necessary to demonstrate the material’s safety for the present medical device. This additional data can be derived from available information on the material itself and thermal effect on the material’s properties.
Vendor data, raw material data, and various certifications help but usually are not 100-percent demonstrative of biocompatibility. Material data only provide assurance that the starting material is safe for its intended use or is within the specification. Vendor data provides a foundation and helps determine where your toxicological assessment needs to focus.
For example, a predicate device may use the same starting material as your device, and the devices may have the same intended use and similar functionality. It would be a mistake to stop here and conclude that, because your device uses the same certified material as the other on-market device, therefore your device is biocompatible. In this case, the fact that the starting material is the same helps narrow your focus to assessing the biocompatibility impact of subsequent manufacturing steps or other areas of your process (e.g., sterilization).
To wit, say a predicate device uses the same starting material as your product; at first glance, that would indicate your product is biocompatible, as well. However, your process may use a chemical (e.g., a cleaning agent) not utilized by the company manufacturing the predicate device. Said chemical may bind to the material surface or prompt a chemical interaction with your product material, whereby it alters the material surface layer’s chemical properties. This chemical change may result in a non-biocompatible material. While this is a hypothetical case, it illustrates the manufacturer’s responsibility to assess from all angles potential impact on biocompatibility.
Another common data source for biocompatibility testing is what I call periphery development work — e.g. the results of cleaning validation and the like. While these data can be helpful to support biocompatibility in a toxicological assessment if utilized correctly, they do not often prove biocompatibility by themselves. Indeed, the degree of detail in those reports often limits their usefulness.
Several problems arise when attempting to leverage a cleaning validation report as a primary justification for biocompatibility. First, cleaning validation often looks at macro data, such as total organic carbon (TOC). Such analysis provides little information from a toxicological assessment standpoint; a TOC value does not provide the level of detail necessary to determine biocompatibility, as different chemicals have different acceptable exposure dosages.
This is true with almost anything: as an extreme case, overconsumption of water can result in water intoxication and there are recorded cases of death due to water intoxication. This does not mean periphery development works cannot be utilized. It simply means that periphery development works alone often provide insufficient proof of biocompatibility but, when combined with other data sources, will help demonstrate biocompatibility in a holistic evaluation.
This leads me to the fundamental aspect of any toxicological / biological safety assessment: it must be viewed through a holistic lens. Both internal and external data can contribute to the overall picture. In addition, data from other applications — for example, safety information on a material used safely in other products — can still be utilized with the appropriate safety factor(s) applied.
When performing a biological safety or toxicological assessment, it is essential that the assessor(s) understand material / chemical safety, as well as the medical device and its manufacturing process. This is because biocompatibility is an element of the finished product; everything from starting materials to the manufacturing process to sterilization will contribute to the finished product’s biocompatibility.
It bears repeating here that good biological safety assessments always frame the assessment result in terms of risk. ISO 10993-1 is risk based and toxicology can never guarantee, with 100-percent certainty, against a biological reaction, as there always exists a potential for outliers.
Novel Materials & Manufacturing Processes
Pivoting to medical devices using novel materials or manufacturing process (e.g., 3D printing), additional considerations may extend beyond ISO 10993-1 Table A1’s recommended endpoints. For example, in 3D-printed medical devices or parts, the support material and curing process used in 3D printing, as well as subsequent chemical treatment to clean and remove the supporting materials, will need to be assessed.
Further, 3D print material often contains additives that facilitate the material’s bonding/curing. These materials are relatively new and unknown, compared to acrylonitrile butadiene styrene (ABS) or polycarbonate parts made through a well-established molding process. As such, additional safety concerns must be considered (e.g., will any leachable be drawn from the part in continual contact with bodily fluid, even if contact duration is less than 24 hours?).
Conclusions
The key takeaway here is that biocompatibility is risk based. While ISO 10993-1 provide guidance on recommended testing, alternative approaches — such as combining predicate device information with other internal and external data — or a mixture of leveraged data and testing can be applied to proving biocompatibility.
Unlike standard biocompatibility testing, biological safety or toxicological assessment is fluid, and its approach varies depending on the available information. Even with the same available information, there may be more than one approach to assessing biocompatibility for your finished product.
Disclaimer: Opinions expressed in this article are those of the author and do not necessarily represent those of the author’s employer.
About The Author
Michael Song, Ph.D., leads the biological device functionality, safety, and digital connectivity group within AstraZeneca’s biologic device development. In his current role, he oversees device functionality and safety, primary container science and technology, biocompatibility, container closure integrity strategy and testing, and digital connectivity development. Prior to his current role, Song was head of the Device, Packaging, and Process Engineering Department at Adello Biologics. He has held key technical positions at Stryker, Amgen, and Kavlico Corporation and has led the development of both combination products as well as 510(k) medical devices. He received his postdoctoral training at Barrow Neurological Institute / St. Joseph Hospital and Medical Center (part of Dignity Health) and holds a B.S. in electrical engineering from Purdue University and a Ph.D. in neuroscience and toxicology from Iowa State University.