Wearable Cardiac Monitoring: Taking The Pulse Of A Thriving Sector
By Tudor Besleaga, Ph.D., and Jacob Skinner, D.Phil., Thrive Wearables
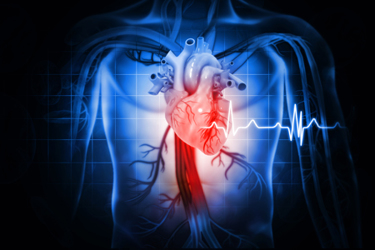
Cardiac monitoring is almost a standard feature of modern wearables but there are still opportunities to get better information and do more with the data. Seizing those opportunities could transform everything from medical care to athletic training and general well-being.
Cardiac monitoring has been around for over 100 years,1 but improved accuracy and wearable technology mean we can now get a better view of cardio performance not only in the hospital but also in the home, at work, or even while the wearer runs a marathon.
Turning this monitoring into actionable insights promises many benefits. Can we determine how well someone is recovering from surgery? Can we measure stress? And can we do so with a system that is low on user friction and high on user value? At present, the most accurate measurements come from the most cumbersome devices, and devices that are comfortable to wear tend not to offer the richest data.
The opportunity for the next generation of products and services is to further blur the distinctions between devices designed for medical professionals, performance athletes, and consumers. We have some obstacles to overcome before we get there, though.
Why Monitor More Than Heart Rate?
It’s worth starting by asking why we want to monitor heart signals with wearables in the first place. It is routine to monitor the heart dynamics of all kinds of patients. The cardiovascular system reveals how a patient is recovering from surgery, the effect of drugs, and more. Sudden changes can indicate serious problems.
Innovation in high-quality cardiac monitoring has also come from devices for athletes. For example, heart rate variability (HRV) – the change in the time between heart beats – and resting heart rate are both indicators of overall fitness, so monitoring of these metrics first appeared in wearables for professional athletes and serious amateurs. These filtered down into more affordable consumer devices pitched as more general lifestyle and well-being assistants.
As well as cardiac monitoring for overall health, some devices can warn of sudden changes in heart rate intervals or test for atrial fibrillation (AFib), a common but potentially dangerous heart abnormality. In a sign of how serious diagnostic tools are being integrated into consumer products, the Apple Watch has been approved as a medical device by the FDA in the U.S. and by other regulators.
ECG Versus PPG
Different use cases will have different ideal technologies and form factors. Essentially, though, wearable cardiac monitors rely on two technologies with different approaches and distinct strengths and weaknesses.
The first is the electrocardiogram (ECG), first developed in the late 19th century. This measures the electrical signal produced by the heart as it beats and is a good guide to heart function. Measurement can be cumbersome, however. A hospital might use a 12-lead ECG, but this restricts movement and needs professional assistance. The consumerization of these devices sacrifices some data resolution but allows them to be worn unobtrusively and comfortably on the wrist for spot measurements instead of continuous ones. In recent years, some smartwatches have added ECG sensors, primarily for detecting things like AFib. These work by sending an electrical signal through the body so the user must wear the watch on one hand and touch the case with the opposite hand to complete the circuit.
Outside the hospital or a testing lab, a chest strap ECG is the best option. This isn’t as accurate as the multiple lead approach but almost everything in this space is a trade-off between user friction and perceived value. The value here is mobility, but the friction comes from reduced accuracy. Moreover, most people don’t want to wear a chest strap for long periods. There isn’t enough value. Cardiologists have tried to get patients to wear all kinds of devices and, other than implanted ones, devices on the wrist are about the only acceptable form factor for most patients.
That works well if the wearer can remember, or be reminded, to perform the ECG regularly but most people would prefer unobtrusive background monitoring. That can be done with a photoplethysmogram (PPG), which was invented in the 1930s and uses a light shone through the skin to track blood flow. This offers insights into cardiac hemodynamics, such as blood pressure trends and cardiac output but also provides localized measurements, such as tissue oxygenation. A downside of this type of sensing is its susceptibility to movement-induced signal loss, particularly at the wrist, where it’s most commonly worn. However, the wrist is a good location for user experience and PPG sensors are now standard in fitness bands and smartwatches, also making the sensor price point increasingly low.
PPG and ECG can complement each other in monitoring the cardiovascular system. The former can be adapted to various form factors and provides long-term continuous measurements, while the latter is limited to chest-based, albeit accurate, continuous measurements and wrist-based spot measurements. It is not uncommon to see PPG watches monitoring alongside chest straps during periods of intense exercise. Or PPG watches using ECG watch spot measurements to obtain quick but reliable and easily comparable readings.
Again, there is a trade-off. A PPG sensor might be most comfortable on the wrist, but the wrist isn’t the ideal place to monitor blood flow. We move our arms, especially when we exercise, which disrupts the signal and means lost data. The system must estimate, or do without, the missing information.
The Next Generation Of Cardio Sensors
Sensors, particularly PPG sensors, are improving all the time, so we can be optimistic about future devices. Most manufacturers have iteratively improved the designs of their PPG sensor modules regularly in recent years, indicating the likelihood of more application options as they improve further.
There are off-the-shelf integrated solutions for measuring PPG or ECG and exporting the data via a serial bus. However, these commonly fall short in signal quality compared to the best in class. Most developers design their own sensor systems, by combining a sensor-body interface, analog front end (AFE), and microcontroller unit (MCU).
The sensor-body interface, which is either an optical front end (OFE) for PPG or electrodes for ECG, is where the most value could be added. The OFE consists of one or more light emitters, commonly LEDs, one or more light sensors, commonly photodiodes (PDs), and a mask to control the light between them. The mask must ensure light passes through the tissue, rather than reflecting straight to the PD, that minimal ambient light enters the sensor, and that good pressure is maintained against the tissue.
The sensor-body interface must be optimized for where it will measure. Red or infrared light is fine for a fingertip PPG, for example, but green light makes data from the wrist less susceptible to motion noise. Some chest devices use both green and red to assess pulses at different depths; the higher the wavelength, the deeper it penetrates.
It is not uncommon for sensors to use discrete components assembled with the mask. However, integrated solutions can save space and cost. These provide PDs with peak sensitivity at the same wavelength as the LEDs and can output three channels (green, red, infrared). One downside is that the distance between the light emitter and the receiver is fixed and typically optimised for wrist cardiac measurement applications. That distance might need to be increased for measuring higher up the arm or at the chest in deeper tissue.
AFEs have improved a lot recently, particularly in signal preprocessing. They can measure ambient light and exclude the baseline, operate LEDs at very low duty cycles, increase energy efficiency, and filter specific frequencies. Some even calculate heart rate intervals for HRV. Many off-the-shelf integrated circuits (ICs) now provide collections of PPG, ECG, or both via multiple channels. When measuring ECG, it is important to select an IC that is optimized for dry electrodes, whether polymer conductive ones, as in chest straps, or stainless steel, as in many watches.
It is important to establish before development how much processing and analysis of the raw signal will be done on the device versus on servers. A lot of memory can be saved if only specific metrics are required, such as HRV, rather than complete raw data.
Since these signals are very susceptible to movement-induced noise, it is common to record accelerometer or inertial measurement unit (IMU) data along with the cardiac data. Motion data can help identify corrupted signal intervals and improve battery life by turning off recordings during high-intensity motion.
For ECG recordings, the MCU will be the main power consumer. Integrated solutions (OFE, AFE, and MCU) do exist. However, they are not quite plug and play, as different form factors require optimization of sensor position and body interface. Furthermore, the onboard MCU means higher energy consumption. They also share limited processed data, as raw sensor data exposes these companies to intellectual property (IP) leak risks.
Medical device manufacturers could benefit from pursuing a hybrid agile approach when developing devices with cardiac sensors, as iterative sensor implementations are to be expected.
Wearable Cardio Monitor Form Factors
One area where PPG sensors show particular promise is in smart earphones, known as hearables. Inside the ear is a good site for a PPG sensor because ambient light interferes less with the reading compared to the wrist. Also of importance is the fact that during exercise, our heads tend to stay stable and the ear sensors are pressed firmly against the inside of the ear. This is particularly promising given the potential for combining hearables with the smartwatch or augmented reality glasses that overlay information about the world onto the lens. As this technology develops, it will make the smartphone less central to everyday information flows for some people.
Some companies, such as Oura, are also adding PPG sensors to rings, which is another part of the body that gives better readings than the wrist. Rings are unobtrusive, and many people like to wear them regardless of their “smart” characteristics. However, this is a difficult product category because the form factor makes it hard to fit in much technology and manufacturers must supply a far greater range of sizes than a watch maker does.
But even if most people continue to rely on wrist-worn devices, there’s reason to expect the sensors to improve. Cardio sensors will continue to get cheaper, smaller, and require less power. That will translate to wearables that are more appealing and more useful in a range of contexts, whether in consumer, professional, elite performance, or medical contexts. The size of the market is expected to reach $4 billion by 2023.
Other sensors will follow the same trajectory, so we will see devices that can monitor blood oxygen levels, breathing patterns, blood pressure, and more. This data, taken collectively, will be combined with heart rate data to create a more accurate picture of overall health. Collecting this data on large groups of people will allow manufacturers to understand how different factors work together. That will mean better algorithms for drawing conclusions from aggregate data and making recommendations in products that increasingly target rare and complex diseases with precision and on the back of huge data insight.
The next challenge is for these developments in the consumer world to feed back into the medical space. Some clinicians still associate wearables with data overload. They are used to taking a single in-clinic cardiac assessment and making decisions accordingly. Switching to real-time, rich data is a change of mindset and requires new training and processes. There is a huge opportunity here, however, to develop a service for clinicians around wearable data. It could transform the way patients are monitored once they leave the hospital.
What might hold that back, however, are the regulatory hurdles and other barriers to entry, such as procurement and support, and changes to healthcare pathways. These have effectively deterred major tech players such as Apple and Google from pursuing this market further. It isn’t easy to create mass market technologies that can support healthcare uses. That does leave the market ripe for smaller players to stake a claim to niche areas. Interestingly, the consumer space for wearables looked similar five to 10 years ago.
Once again, we come back to reducing friction and increasing value. There is tremendous value in quality cardiac monitoring with actionable insights, for patients, athletes, and consumers. If we can break down the barriers of existing health provision, educate doctors and health providers, and deploy in-home and long-term monitoring, then we will start to break through into better health and wellness for huge numbers of people. We have only just begun to understand what is possible.
About The Authors:
Tudor Besleaga, Ph.D., is Thrive Wearables’ sensor systems engineer and a project lead on cardiovascular health research. So far at Thrive, he has worked on solutions that non-invasively measure blood oxygenation, breathing, heart rate, heart rate variability, and skin and core body temperature. He holds a Ph.D. in biomedical engineering, and his background is in multi-disciplinary teams across commercial, research, and healthcare organizations. Tudor has conducted data collection projects from protocol design to device development and data analysis, has experience in 3D modeling and programming from chip to high level languages, and has expertise in signal processing and algorithm development for biomedical systems focused on vital signs.
Jacob Skinner, D.Phil., is CEO of Thrive Wearables. He has worked with human-centred technology for 10 years. Jacob’s passion lies in using technology within health and wellness, and his mission for Thrive is to achieve exponential gains in this space with the creation of data-driven, human-centred wearable technology products. Having completed a D.Phil. in experimental physics, Jacob has designed commercially available electrophysiology sensors and applications in the Sensor Technology Research Centre at the University of Sussex and has secured multiple InnovateUK, NIHR, and other grant funding.