Plastics Selection For Medical Wearables: Key Material Families
By Eric Larson, Art of Mass Production
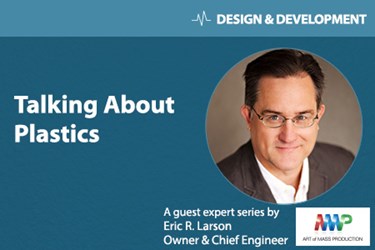
This article is the third in a three-part series. You can read part one here, and part two here.
The process of material selection often begins by evaluating the characteristics of a given polymer family versus other polymer families. This evaluation is done in concert with a thorough review of the performance requirements of the device for which the materials are intended. Once a suitable material family is found, designers must determine the exact grade and version that is suitable (including which additives are required) for their product, as well as source an appropriate material supplier.
Below is a short list of polymer families that could be considered for use in a medical wearable, either for housings, fittings, fasteners, or connectors. Each family has advantages and disadvantages. Furthermore, within each family, there are numerous grades and versions (variations) available, including FDA-approved grades and versions with additives for structural reinforcement (via glass fiber, mineral, etc.), UV stability, and/or processing improvements.
Acrylonitrile butadiene styrene (ABS) is a key engineering material, suitable for a variety of applications. A terpolymer (a polymer made from three separate monomers), the name is derived from the monomers: A - acrylonitrile (a vinyl monomer), B - butadiene (rubber), and S – styrene. LEGO blocks (at right) are made from ABS.
ABS offers good mechanical properties, including a balance of strength, stiffness, and toughness. Numerous grades are available, including those specially formulated for super-high impact, with excellent low-temperature toughness. The material properties and raw material cost vary depending on the exact version, which usually is determined by the ratio of the three polymers. By default, ABS is a very high-gloss material because of the styrene component. Thus, it is an excellent choice for consumer applications, and it frequently is used in housings for consumer electronics, consumer appliances, and general purpose power tools. Solvent resistance is fair to good.
Acrylonitrile styrene acrylate (ASA) is another terpolymer, and its name is also derived from the monomers: A - acrylonitrile (a vinyl monomer); S – styrene; and A – acrylate. You may recognize the term acrylate from the polymer Polymethyl methacrylate (PMMA), commonly known as acrylic.
ASA is similar to ABS; one might even call them polymer brothers. Again, the material properties vary depending on the version, again usually determined by the ratio of the three monomers. One key advantage of ASA over ABS is its excellent UV resistance, giving it better long-term performance in outdoor applications, and greater resistance to aging and yellowing.
However, be aware that some versions of ASA can be a little soft (low hardness) — not as soft as a polyethylene, but definitely softer than polycarbonate or a general-purpose ABS. Such low-hardness ASAs can be more susceptible to marring and abrasion.
Acetal, also known as polyoxymethylene (POM), polyacetal, and polyformaldehyde, is a semi-crystalline thermoplastic with an interesting mix of properties. First, it has excellent mechanical properties, including high strength, stiffness, and toughness. Second, it is resistant to a variety of chemicals – a result of its crystalline structure. Third, acetal has unique characteristics of tribology – the science of friction, lubricity, and wear. It has a very low coefficient of friction and outstanding wear characteristics, all of which can be further enhanced with additives, such as chemical lubricants and PTFE. The combination of these characteristics make acetal ideal for many applications, including use as tabs on lighters (pictured at right).
Acetal also has exceptional knit-line strength, approaching 100 percent of the knit-line strength of the base material, in some instances. This means that you can design and mold extremely complex parts with high structural integrity (just try doing that in ABS). These traits combine to make acetal an easy material to machine. It therefore is great for making prototypes. It also has a very pleasing surface appearance, regardless of whether it is molded, extruded, or machined.
Note however, that acetal, compared to other thermoplastic materials, has a very high level of crystallinity (75-85 percent). This gives the material its strength, stiffness, and resistance to chemical attack. It also means that molded acetal has a high amount of mold shrinkage. So, one needs to plan for this in design and tooling decisions.
Polycarbonate (PC) is an amorphous thermoplastic with a fantastic balance of properties. It is stiff, strong, has good ductility, and can be made in clear form (with light transmission comparable to glass). But, in my mind, what makes polycarbonate unique is its exceptional impact resistance. While it does have some notch sensitivity (as do most thermoplastic materials), in solid or sheet form the impact resistance of polycarbonate is unmatched. As an example, thermoformed polycarbonate is used for the cockpit canopy of several fighter jets, including the F-22 Raptor and the British Hawk T1 training aircraft pictured (at right). If your application involves any kind of impact, polycarbonate should be on your short list of materials to consider.
I often think of polycarbonate by comparing it to other materials: “It’s kind of like acrylic, but with better physical properties (and it’s more expensive).” “It has a balance of properties like ABS, but with better toughness (and it can be made clear).”
Polyamides (PA) are a family of polymers, the generic name for which is “nylon.” These polymers contain chains of monomers that are bonded together via amide bonds, hence the term “polyamide.” Nylon can be fabricated as a co-polymer, with two different types of monomer, or as a homo-polymer, using a single monomer. The type of nylon is designated by the number of carbon atoms in the base monomers. The most common types are nylon 6 and nylon 6/6, but there also are more specialized types, including nylon 11, nylon 12, nylon 5/10, nylon 6/12, et al.
Nylon has some unique characteristics: First, it is a semi-crystalline material, and like most semi-crystalline materials, has excellent chemical resistance. Second, it has a relatively high melting point, and thus is able to withstand high end-use temperatures. Third, it inherently has a great deal of lubricity, and excellent resistance to abrasion and wear. Fourth, nylon absorbs moisture, either from the atmosphere, or directly from contact with water, which affects its physical properties (making it tougher and more flexible), and overall dimensions. The amount of moisture in the material is dependent on the relative humidity.
Added together, polyamides offer an unparalleled combination of strength, stiffness, and elongation. One application that demonstrates this succinctly is a standard cable tie. The tail end of the tie provides the structure, and also has an angled gear rack molded into it. The head of the tie contains a flexible ratchet. This ratchet flexes to allow the tail to be inserted, and then flexes click-click-click over the gear rack as the tie is tightened. But, if the tail is pulled backward, the ratchet locks in place, and the tail cannot be removed.
Polyester, as a term, describes a number of polymers that contain an ester group in the polymer chain. This includes a wide range of materials, including organic and synthetic materials, and thermoset and thermoplastic resins. For design engineers, there are two important thermoplastic polyester resins: polyethylene terephthalate (PET), and polybutylene terephthalate (PBT). Both of these resins are homopolymers, are typically thought of as engineering resins, and are often used with glass fiber reinforcement for structural applications. However, PET also can be used in the form of a fiber, a film, or as a packaging resin (e.g., PET bottles).
In addition, PET can be modified into a number of copolymer forms. Often called copolyesters, common forms include PETG, PCTG, PCTA, and PCT. Copolyesters offer good mechanical properties and chemical resistance. Since they also can be made in a clear form, they have an advantage over polycarbonate because of their superior chemical resistance.
Alloys and Blends
One of the most interesting aspects of thermoplastic materials lies in their ability to be modified. While this often is accomplished through the use of additives, and it also can be done through the use of another thermoplastic material. It is quite common to mix one or more thermoplastic materials in the lab, during the resin production process, or even on the factory floor. The mixing can be done by combining solid pellets (commonly known as cube blending), or by mixing materials while they are in a molten state (either via melt blending or compounding). The resulting mixed material is known as a resin blend.
As a result of this mixing, one of several things can happen. Sometimes, the materials do not mix together well. In such cases, they probably are not chemically compatible. If you tried to mold parts from this mix, you would likely end up with a swirled-looking part that separates at the boundaries between the materials. However, if the materials are compatible, they will mix together easily, with no discernable boundaries between where one material starts and the other begins. The resulting blended material typically has properties that are midway between the two base materials.
Two of the more interesting blends for wearable devices are PC/ABS blends and PC/polyester blends. In either case, the polycarbonate component contributes its toughness characteristics, and the other component helps reduce the overall cost.
Another result of mixing two materials — and in many ways the preferred result — is that the two materials will not only have a chemical affinity for one another, they will combine to form a new material with properties greater than the sum of the individual components. This is a thermoplastic alloy. Unlike a simple blend, production of a thermoplastic alloy typically involves more than just mixing, and such alloys usually are available only from resin suppliers and specialty compounders. The portfolio of available alloys is virtually unlimited, although not all of them are useful in the design of a wearable device.
Evaluating Materials
As we have discussed, the material requirements for a wearable device are numerous and complex. Often, there will be one or more requirements that stand out (such as optical transparency, or resistance to a specific chemical), and these requirements will effectively filter the list of material families, a process of material de-selection. From there, one can then look at the remaining families in greater detail, evaluating the suitability of materials in those families, and refining the need for additives as the development process progresses. We will look at the use of thermoplastic elastomers in the next part of this series.
Further Reading
- Story, M.F. (2010). Medical Devices in Home Health Care. In National Research Council, The Role of Human Factors in Home Health Care: Workshop Summary, Olson, S, Rapporteur. Committee on Human-Systems Integration, Division of Behavioral and Social Sciences and Education. Washington, DC: The National Academies Press.
- Rubin, J. and Chisnell, D., (2008). Handbook of Usability Testing: How to Plan, Design, and Conduct Effective Tests. New York: John Wiley and Sons, Inc.
- Dumas, J. and Loring, B. (2008). Moderating Usability Tests: Principles and Practices for Interacting. San Francisco, CA: Elsevier/Morgan Kauffman Publishers.
- Larson, Eric R. (2015). Thermoplastic Material Selection: A Practical Guide. Oxford, UK: Elsevier/William Andrew Publishers.